Revolutionizing Electronics: Bismuth Oxide Film Stabilizes Ferroelectricity down to 1 nanometer
This research presents a breakthrough in the field of atomic-scale ferroelectrics by demonstrating the stability of ferroelectricity in a layered film of bismuth oxide down to 1 nanometer through samarium bondage. The research findings have been published in the journal Science, where Linxing Zhang from the University of Science and Technology Beijing and Yue-Wen Fang from CFM led the experimental and theoretical work, respectively.
Ferroelectrics exhibit spontaneous electric polarizations that can be reversed by an external electric field. Thy have been applied into information storage, sensors and ultrasound devices. Nowadays, the ferroelectric industry is valued at approximately $7 billion and continues to grow. The CFM researcher Dr. Yuewen Fang has collaborated Dr. Linxing Zhang at the USTB to design a new ferroelectric material that pushes the size down to the atomic scale. The work has been published today in Science.
The material, which consists of a layered structure of bismuth oxide stabilized by samarium bonding, shows a remanent polarization of up to 17 microcoulombs per square centimetre even at thicknesses as small as 1 nanometer. It has a high Curie temperature of approximately 500 K, making the room-temperature applications possible.
The crystal structure prediction was primarily led by CFM and involved a collaboration with Dr. Oswaldo Dieguez at Tel Aviv University. The theoretically proposed crystal structure is in a good agreement with the cross-sectional high-angle annular dark-field scanning transmission electron microscopy images observed in different directions, implying the accuracy of the crystal structure prediction (see Figure 1).
The first-principles calculations revealed that the ferroelectricity is driven by the lone-pair electrons of Bi ions. These electrons are resided in one side of Bi ions asymmetrically, which breaks the inversion symmetry and induces the ferroelectricity. In addition, the study also finds that the doping of samarium into the sample is crucial for the thermodynamic stability of the polar structure especially when the size is reduced to 1 nanometer, according to the ab initio molecular dynamics simulations by Dr. Fang.
Overall, these ultrathin ferroelectric films are highly suitable for future nano-electronic devices, particularly in the areas of field-effect-transistors, low-power logic, and non-volatile memories. The structure design of these films has enormous potential for manufacturing atomic-scale electronic devices. In fact, the team has achieved a giant tunnelling electroresistance of 700,000 in the atomic-scale ferroelectric tunnel junctions based on the above material very recently (See Nature Communications).
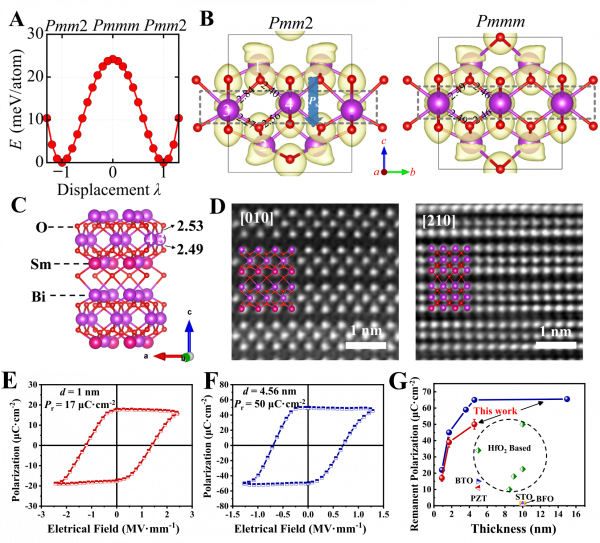
Figure 1: The proposed crystal structure and the interpretation of ferroelectricity. (A) The computed ferroelectric transition between the polar and non-polar structures. (B) The electron localization function of the two phases demonstrating the role of long-pair electrons of Bi in driving the ferroelectricity. (C) The Sm doped Bi6O9 structure in which the polar symmetry is preserved. (D) The comparison between the theoretical structure and the HAADF-STEM images. (E and F) The polarization hysteresis loops of the films with thicknesses of 1 nm (E) and 4.56 nm (F). (G) The comparison with other ferroelectric systems in remanent polarization.
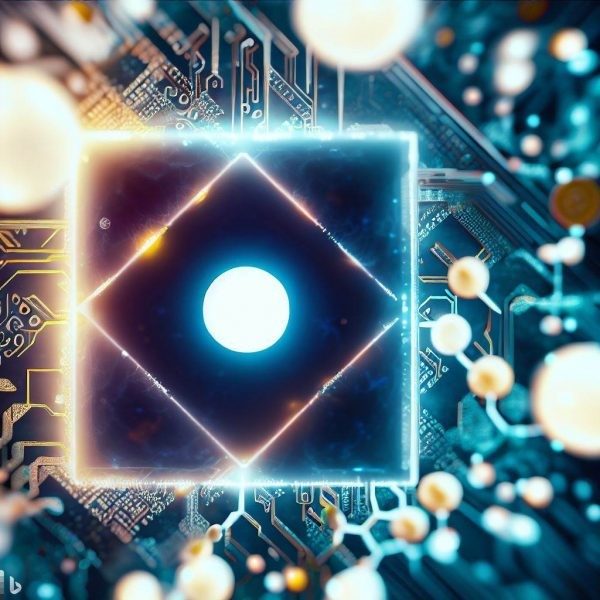
Figure 2. The future subnanometer untrathin memory devices based on atomic scale ferroelectrics.