Cation Dynamics and Structural Stabilization in Formamidinium Lead Iodide Perovskites
Organometal halide perovskites are unique hybrid (organic-inorganic) materials, as well as one of the most promising candidates for the development of new and sustainable photovoltaic and photonic technologies. At present, the primary barrier hindering their widespread use relates to their hard-to-control polymorphism and poor physico-chemical stability, making the discovery of new and improved target materials a costly and slow trial-and-error endeavor.
Addressing the aforementioned challenges requires a robust and coherent understanding of the microscopic (atomic-scale) mechanisms underpinning the stabilization of the photophysically active phases of these hybrid media, a challenge to both experiment and theory. In this particular work, state-of-the-art neutron spectroscopy and computational materials modelling have been combined to study the structural stabilization of the hybrid perovskite formamidinium (FA) lead iodide (FAPbI3), via chemical doping with methylammonium (MA) cations. As starting point, we have conducted for the first time an exhaustive analysis of FA-cation motions and underlying local structure around the organic moiety within the inorganic perovskite cages. Detailed validation against the experimental data provides unequivocal evidence for the formation of an orientational glass responsible for phase metastability in FAPbI3. This analysis also constitutes a suitable starting point to explore the stabilization of the perovskite framework via doping with MA cations. In particular, we find that hydrogen-bonding motifs involving directly the FA cations are strengthened as a result of cage deformation upon doping, as shown in the right panel of the Figure. This synergistic ´locking´ effect across perovskite cages is driven by librational motions, and it is accompanied by a concomitant weakening of MA interactions with the surrounding framework. Such stabilization mechanism is promoted by the inherent softness of the lattice, along with a strong overlap of characteristic low-energy modes of inorganic and organic counterparts. In addition to unveiling a hitherto unknown mechanism of structural stabilization in this class of materials, this work also brings to the fore the pivotal role played by dynamical disorder as a primary (and quite often overlooked) driver of phase behavior.
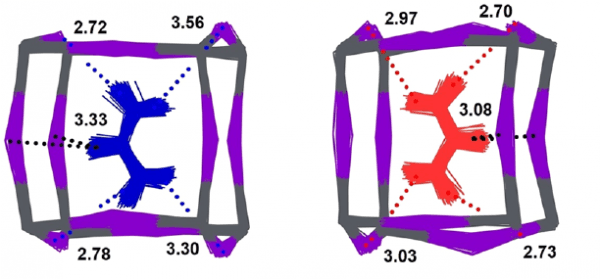
Figure: FA-cation configurations in FAPbI3, corresponding to weak (left, blue) and strong (right, red) hydrogen bonding with the inorganic cage, the latter case as a result of chemical doping. Close contacts between organic-cation hydrogens and iodine are given in Angstrom.