Vibron-assisted spin excitation in a magnetically anisotropic molecule
The Scanning Tunneling Microscope (STM) drives electronic currents through a very small spatial region, allowing the excitation of single atoms and molecules. When an excitation is produced, the current increases because there are more transmission channels for the passing electrons. Then, by recording the current as a function of the applied bias between the tip of the STM and the surface, it is possible to obtain information on the molecule.
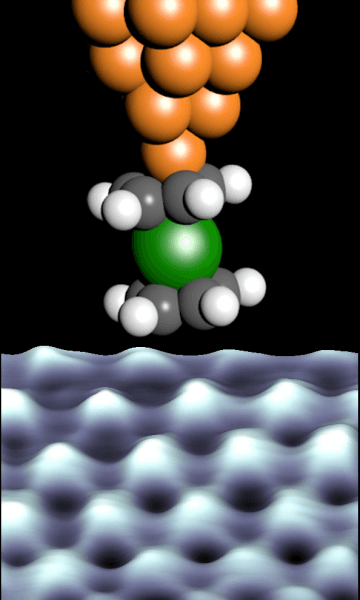
A nickelocene molecule (C10H10) trapped by an atom of the STM tip over a surface.
In this work, the authors use nickelocene molecules (see the figure). This molecule is magnetic and anisotropic. This means that the molecular spin does not have complete symmetry. As a consequence, there are two degenerate excitations taking place at 3.8 meV and they correspond to turning the molecular spin by 90 degrees.
In the present work Bachellier et al. show that the molecule can also have vibrational excitations, and when the electrons have enough energy to simultaneously excite vibrational and spin degrees of freedom, they do it with an enormous efficiency. This is really surprising because inelastic electron transmission is usually thought to be less efficient than the corresponding elastic process, let alone to have two simultaneous excitations of the spin-excitation signal.
The authors have rationalized these findings in terms of the high efficiency of the spin excitation. It happens so likely that it is not deterred by the excitation of the vibration, leading to a very important spin+vibration signal. From the theory point of view, the results were equally puzzling. It was necessary to use a many-body formulation of the problem to be able to capture the excitation process. The model Hamiltonian contains electronic correlations that allow obtaining clear spin excitations by changing the electronic configuration. The surprise comes when an electron-vibration interaction is added to the Hamiltonian. Automatically the experimental result is recovered, not only opening the vibrational excitation, but also the vibrational and spin combined excitation. This is a surprise because there is no direct vibration-spin interaction in the Hamiltonian, but as in the experiment, the strong electron correlation suffices to create it.
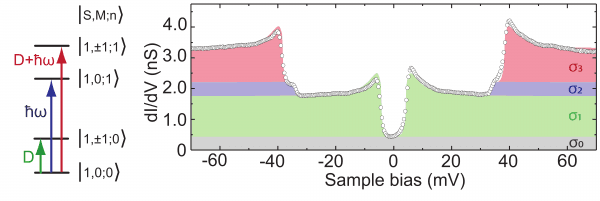
(LEft) Energy levels with spin and vibrational degrees of freedom. D is the magnetic anisotropy equal to 3.8 meV and the frequency (ħω) is equal to 34 meV. (Right) Differential conductance over the nickelocene adsorbates showing the first rapid increase at 3.8 mV, a second shoulder at 34 mV and the combined excitation at 38 mV.